Drug Nano-Carriers Marching towards Process Intensification
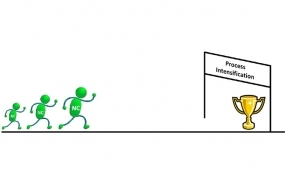
Did you know, "...An estimated 128,000 new cases of cancer will be diagnosed in Australia this year, with that number set to rise to 150,000 by 2020." [1]
In addition, "...Cancer is a leading cause of death in Australia - more than 43,000 people died from cancer in 2011 - about 3 out of 10 deaths in Australia." [1]
The increasing number of people diagnosed with cancer in Australia alone has given rise to alternate drug delivery methods, in particular targeted drug delivery using nano-carriers. This method has grown more popular in treating cancers and tumours.
The application of nano-carriers has been used in the therapy of cancer treatment including chemotherapy agents, antibiotic, antifungal, antiprotozoan, influenza vaccine, hepatitis vaccine, analgesia, immunotherapeutic, and many more.
Commercially available drug nano-carriers and others that are still being researched have been reported in a review paper titled, Lipids-based drug carrier systems by dense gas technology: A review. [2].
PrinSus Research Group in the School of Chemical Engineering at UNSW Australia (previously known as Supercritical Fluid research group) has developed a batch dense gas process known as ‘depressurization of an expanded solution into aqueous media’ (DESAM) and a novel continuous dense gas process, known as ‘nano-carrier by a continuous dense gas’ (NADEG). These processes have been created to produce nano-carriers for pharmaceutical applications.
Nano-carriers such as liposomes, polymersomes and micelles have been successfully produced by both the DESAM and NADEG processes. These nano-carriers are well known for their role in transporting Active Pharmaceutical Ingredients (APIs) within the body, through a delayed and targeted controlled release of APIs. This allows greater control over dosage and release rate of drugs, increase of therapeutic efficacy and minimisation of side effects.
The above-mentioned nano-carriers are all self-assembled nanostructures made of amphiphilic molecules (Fig 1). Liposomes and polymersomes are membrane vesicles containing an aqueous inner core. Their constituents (Fig 1) self-assemble into bilayer membranes (Fig 2) and eventually form vesicles (Fig 3) when contacted with water. They are able to carry hydrophilic compounds in the aqueous domain while hydrophobic compounds can be hosted within the membrane, as illustrated in Fig 3. Micelles self-assemble into single layer membrane with a hydrophobic domain as the inner core. Hence, micelles can only carry hydrophobic compounds, as shown in Fig 4.
The Problem
Several conventional methods for production of nano-carriers have limitations such as time consuming, complex procedures, harsh operating conditions and use of large amounts of toxic organic solvents. Additional procedures required to thoroughly remove residual organic solvent is essential because organic solvents present occupational and environmental risks, and are not ideal for pharmaceutical applications. Furthermore, conventional methods have poor control over the formation of nano-carriers and their morphologies.
PrinSus Research Group applies the dense gas technology to the formation of nano-carriers to address these limitations. Implementing the dense gas technology to innovate the production techniques of nano-carriers in effective and environmentally acceptable ways.
Towards Process Intensification
The Solution
A batch dense gas technique known as ‘depressurization of an expanded solution into aqueous media’ (DESAM) was developed to produce liposomes in one-step at moderate temperatures and pressures [3]. The DESAM process uses a dense gas to pre-expand an organic solution that contains the raw materials, which is subsequently introduced into an aqueous media at atmospheric condition by the pressure gradient via a nozzle. The DESAM process was then further investigated to produce other nano-carriers – polymersomes and micelles, and drug encapsulated vesicles (liposomes and polymersomes) [4, 5]. Both residual solvent removal and encapsulation studies by the DESAM process were proven better than the conventional production methods [3, 6-11]. A schematic diagram is illustrated in Fig 5.
A novel continuous dense gas process, nano-carrier by a continuous dense gas (NADEG) process has been developed as an evolution of the batch DESAM process [12]. The main advantage of using a continuous process over a batch process is the increase in production rate while lowering labour demand. The NADEG process was introduced because it has better control over the formation of nano-carriers and reduction of residual solvent levels than the DESAM process. In the NADEG process, a low volume mixing tee (LVMT), as illustrated in Fig 6, was used to achieve better mixing during the formation of nano-carriers when the raw material solutions and an aqueous solution come in contact, in comparison to the DESAM process. The resulting suspension is then delivered to a packed bed from the top of the vessel with supercritical carbon dioxide being introduced from the bottom of the vessel to achieve an efficient organic solvent extraction. A schematic of the NADEG process is shown in Fig 7.
The NADEG process is effectively a 4 times scale up of the batch DESAM process, which is a leap forward to process intensification. In addition, the NADEG process has the ability to generate products free from residual solvent [12]. More details about the NADEG process can be found at: http://dx.doi.org/10.1016/j.cej.2014.12.072
The differences between the DESAM and NADEG processes are summarised in table below:
Reference
[1] Facts and figures, in, Cancer Council Australia, 2014.
[2] C.C. Beh, R. Mammucari, N.R. Foster, Lipids-based drug carrier systems by dense gas technology: A review, Chemical Engineering Journal, 188 (2012) 1-14.
[3] L.A. Meure, The development of a novel process for the formation of liposomes: Depressurisation of an Expanded Solution into Aqueous Media (DESAM), in: Chemical Sciences and Engineering, The University of New South Wales: Sydney, 2004.
[4] C.C. Beh, Formation of nano-carrier systems by dense gas processing, in: School of Chemical Engineering, UNSW Australia, Sydney, Australia, 2013, pp. 225.
[5] C.C. Beh, R. Mammucari, N.R. Foster, Formation of Nanocarrier Systems by Dense Gas Processing, Langmuir, 30 (2014) 11046-11054.
[6] R. Pandey, S. Sharma, G.K. Khuller, Liposome-based antitubercular drug therapy in a guinea pig model of tuberculosis, International Journal of Antimicrobial Agents, 23 (2004) 414-415.
[7] J. Lasch, V. Weissig, M. Brandl, Preparation of liposomes, in: V.W. Torchilin, V. (Ed.) Liposomes: A practical approach, Oxford University Press, 2003, pp. 3-29.
[8] R.C.C. New, Preparation of liposomes, in Liposomes: A Practical Approach, 1990.
[9] D.W. Deamer, Preparation and properties of ether injection liposomes, Annals of the New York Academy of Sciences, VOL. 308 (1978) 250-258.
[10] D. Deamer, A.D. Bangham, Large volume liposomes by an ether vaporization method, Biochimica et Biophysica Acta (BBA) - Biomembranes, 443 (1976) 629-634.
[11] F. Szoka Jr, D. Papahadjopoulos, Procedure for preparation of liposomes with large internal aqueous space and high capture by reverse-phase evaporation, Proceedings of the National Academy of Sciences of the United States of America, 75 (1978) 4194-4198.
[12] C.C. Beh, R. Mammucari, N.R. Foster, Process intensification: Nano-carrier formation by a continuous dense gas process, Chemical Engineering Journal, 266 (2015) 320-328.